Create AI-powered tutorials effortlessly: Learn, teach, and share knowledge with our intuitive platform. (Get started for free)
Independent Assortment Breaking Down Mendel's 7 Key Cross-Breeding Experiments with Pea Plants
Independent Assortment Breaking Down Mendel's 7 Key Cross-Breeding Experiments with Pea Plants - Stem Height Experiment Revealing 3 to 1 Dominance Pattern in F2 Generation
Mendel's experiment focusing on stem height in pea plants yielded a notable 3:1 ratio in the second filial generation (F2). Three-quarters of the F2 plants exhibited the tall stem trait, characteristic of the dominant allele, while the remaining quarter displayed the recessive dwarf trait. This consistent pattern strongly supports Mendel's Law of Segregation. This law proposes that during the creation of reproductive cells (gametes), the paired alleles governing a specific trait separate, leading to each gamete carrying only one allele. The F2 generation's outcome provides empirical evidence for this process. Furthermore, it reinforces the idea that dominant traits tend to manifest more frequently than recessive traits in subsequent generations, a cornerstone principle in understanding how inherited characteristics are passed down. His careful observation of these patterns was pivotal in establishing the foundations of genetics, underscoring the crucial role of dominant and recessive alleles in determining trait expression.
When Mendel crossed tall and dwarf pea plants, the first filial (F1) generation exclusively displayed the tall phenotype, suggesting a dominant trait. However, the intrigue emerged in the subsequent F2 generation. Here, a consistent 3:1 ratio of tall to dwarf plants appeared. This 3:1 ratio strongly indicates the presence of a dominant allele masking the expression of a recessive allele for stem height. This is the core idea of Mendelian dominance – a single allele can dictate the phenotype, even if other alleles are present.
Mendel's choice of pea plants proved insightful. Their rapid generation time and capacity for self-pollination allowed for swift and accurate tracking of trait inheritance across multiple generations. This efficiency provided a clearer picture of the inheritance dynamics, particularly the unpredictable reappearance of recessive traits in the F2 generation.
The observed ratio implied a fundamental idea: tallness had a stronger influence over the plant's phenotype compared to dwarfness. This concept was a cornerstone in demonstrating that traits could separate and assort independently during reproduction. In this case, the allele for tallness segregated independently from the allele for dwarfness, influencing how these characteristics were expressed in the offspring. This understanding forms the basis of the principle of segregation.
Furthermore, his rigorous approach controlled pollination and also captured the remarkable variability in plant height within the F2 generation. This emphasized the complex nature of heredity, moving beyond simplistic dominance hierarchies. While the idea of a 3:1 ratio is seemingly simple, it points to a far deeper complexity in the dynamics of genetic inheritance than initially envisioned.
The experiments demonstrated the ability to create pure-breeding lines, enabling meticulous control over the genetic background. This practice was crucial to the methodology, making it possible to attribute any observed phenotypic variations directly to the intended inherited traits. Without this, the conclusion on this 3:1 dominance pattern would have been far less certain.
This pattern puzzled many of Mendel’s contemporaries. They struggled to reconcile how a trait could seem to vanish in one generation and then reappear in the next. This very phenomenon later found explanation through Mendel’s understanding of how alleles segregate during the formation of gametes, providing a clearer understanding of inheritance patterns.
The quantitative approach to analyzing plant heights and their ratios was pioneering for its time. By employing ratios as a metric, Mendel helped lay the foundation for future genetic studies heavily reliant on statistical analyses. This quantitative element ushered in a new era of biological analysis where numbers played a crucial role.
Though the implications of Mendel's findings were initially overlooked, they are widely acknowledged today. It took several decades for scientists to realize the profound implications of his discoveries. Once recognized, it led to the rise of modern genetics as we know it today, highlighting the importance of methodical work and delayed recognition of seminal research findings.
Interestingly, these traits for stem height are known to reside on different chromosomes, illustrating the critical principle of independent assortment. This phenomenon reveals that the inheritance of one trait doesn't influence the inheritance of another. This is fundamental for our understanding of how multiple traits can be expressed in different combinations within a single organism.
Mendel's stem height experiment provided the very basis for exploring the mechanisms of genetic linkage and chromosome behavior. It offered a framework for investigating far more complex genetic relationships, demonstrating that patterns in simpler systems can offer insights into more complex biological scenarios. His contribution extended beyond a simple ratio; it unveiled the fundamental building blocks of heredity and offered a launching point to dissect the complex world of genetics that still fascinates researchers today.
Independent Assortment Breaking Down Mendel's 7 Key Cross-Breeding Experiments with Pea Plants - Flower Color Testing Demonstrating Complete Dominance of Purple over White
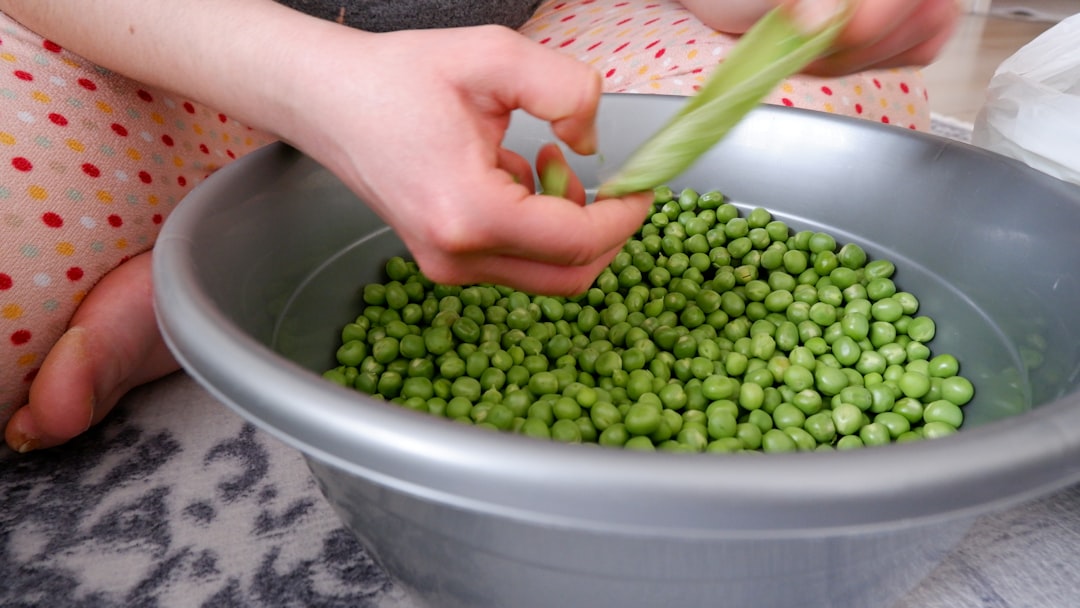
Mendel's investigation into flower color involved crossing purple and white flowering pea plants. The resulting first generation (F1) offspring consistently displayed purple flowers, revealing that purple flower color completely dominates over white. This dominance is reflected in the genetic makeup of the plants where purple can be either homozygous dominant (FF) or heterozygous (Ff), while white flowers are always homozygous recessive (ff). Essentially, the presence of even one dominant purple allele masks the expression of the white allele. This demonstration of complete dominance was a key piece of Mendel's work, forming the basis for his broader laws of inheritance, namely the Law of Segregation and the Law of Independent Assortment. His findings about how alleles interact to determine observable traits are foundational to modern genetics. This early exploration provided deep insights into how traits are inherited and has become a cornerstone of our understanding.
1. **The Genetics of Flower Color**: Mendel's initial focus on flower color in pea plants revealed a straightforward dominance relationship. The purple flower color consistently masked the white, indicating that a single gene could control the visible trait. This simple observation highlights how dominant-recessive patterns play a crucial role in inheritance.
2. **Beyond Genes: Environmental Influence**: While purple flower color is genetically dominant, environmental factors, such as soil pH, can impact flower pigmentation. This illustrates that the visible trait (phenotype) can be shaped by external conditions, even when the genetic makeup favors a specific outcome.
3. **Dominant Traits & Recessive Potential**: The clear dominance of purple over white not only demonstrates Mendel's Law of Dominance but also emphasizes how recessive traits, though hidden in some generations, can persist in the genetic makeup. These traits might reappear in future generations under different environmental conditions or breeding combinations.
4. **Foundation for Genetics**: The observation of complete dominance in flower color experiments acts as a fundamental building block for understanding more complex inheritance patterns. This simplicity is vital as researchers explore traits controlled by multiple genes or those influenced by the environment.
5. **Pea Plants: A Strategic Choice**: Mendel's choice of pea plants, with their distinct and easily observable flower colors, highlights the importance of selecting appropriate model organisms in genetic research. This approach, still relevant today, ensures researchers can clearly observe and interpret results in genetic studies.
6. **The Impact of Dominant Alleles**: While the dominant purple allele dictates flower color in Mendel's experiments, the recessive allele for white flowers retains the potential to reappear. This necessitates careful tracking of alleles across generations, especially when attempting to predict the emergence of certain traits in breeding experiments.
7. **Alleles and Their Role**: The purple and white flower colors are controlled by different alleles at the same gene location (locus). This fundamental concept of allelism is critical for grasping inheritance mechanisms. It forms the basis of understanding genetic mapping and various contemporary breeding techniques.
8. **Quantifiable Ratios in Inheritance**: The consistent 3:1 ratio of flower colors observed in the F2 generation showcases the quantitative nature of Mendelian genetics. This ability to measure outcomes and predict ratios has greatly influenced breeding and hybridization efforts across species.
9. **The Art of Controlled Cross-Pollination**: Mendel’s meticulous cross-pollination technique ensured the reliability of his flower color data. This level of experimental control in hybridization practices has become a cornerstone for modern plant breeding and agricultural practices.
10. **The Language of Genetics**: The standard genetic notation (e.g., using "P" for purple and "p" for white) represents dominant and recessive alleles. This convention aids in visualizing gamete combinations and predicting inheritance patterns, facilitating genetic research across various organisms.
Independent Assortment Breaking Down Mendel's 7 Key Cross-Breeding Experiments with Pea Plants - Seed Shape Analysis Tracking Round vs Wrinkled Inheritance Patterns
Mendel's study of seed shape in pea plants, specifically the contrast between round and wrinkled seeds, provided critical insights into dominant and recessive traits. Round seeds were the dominant trait, consistently appearing in the first generation (F1) of crosses. However, the recessive wrinkled seed trait reappeared in a predictable pattern in the following generation (F2). This clear distinction in seed shape reinforces Mendel's principles of segregation and independent assortment, showing that these traits separate independently during the creation of reproductive cells. The dominant round trait masked the recessive wrinkled trait, emphasizing the concept of dominant allele expression, a foundational aspect of modern genetics. By analyzing the inheritance of seed shape, Mendel not only further developed our understanding of heredity, but he also created a basis for future investigations into genetic variation and its effects. His findings served as a starting point for unraveling the intricacies of genetics.
Mendel's exploration of seed shape in pea plants, specifically the contrasting traits of round and wrinkled, offered a fascinating window into inheritance patterns. The round seed shape (R) exhibits complete dominance over the wrinkled shape (r), resulting in a familiar 3:1 phenotypic ratio in the second filial generation (F2). However, the persistence of the recessive wrinkled trait in the F2 is a compelling reminder of the underlying genetic diversity.
The difference in seed shape boils down to variations in starch composition. Round seeds store more amylopectin, yielding a smoother texture, while wrinkled seeds possess a higher concentration of amylose, leading to a shriveling effect as they lose moisture. This difference in starch type, and its effect on water retention, may surprisingly provide ecological benefits to wrinkled seeds in drier environments. This exemplifies how a seemingly simple, visually recessive trait can have a hidden advantage.
The seed shape experiment highlights the elegance of Mendel's principles, particularly segregation and independent assortment. During meiosis, the pairing of R and r alleles separates, creating a variety of potential genetic combinations in the resulting gametes. This is foundational to how traits are passed to the next generation.
His rigorous methodology of meticulously tracking the R and r alleles across generations was a major innovation in genetics. Establishing pure-breeding lines was critical to isolate the impact of specific traits and minimize extraneous variables. These practices, while perhaps laborious, proved essential for making accurate conclusions about inheritance patterns.
Furthermore, seed shape was a perfect visual model to study because the variations were so distinct and easy to observe. This simplicity underscored the notion that even simple traits could offer powerful insights into complex genetic concepts.
It's important to remember that while the round and wrinkled seed shape analysis appears simple, it represents a foundational block, but by no means represents the whole picture of genetic complexity. Many traits are influenced by multiple genes and their interaction can be incredibly intricate.
Interestingly, the concept of recessive traits initially confused many scientists at the time. Their struggle to grasp why traits could seemingly disappear and then reappear led to a delayed acceptance of Mendel's work. His elegant explanation using allele segregation finally provided clarity, showcasing the importance of thorough experimental work and recognizing the sometimes delayed recognition of insightful findings.
Mendel's seed shape analysis laid the groundwork for quantitative genetics. The ability to analyze and quantify seed shape ratios across generations underscored the significance of statistical approaches in studying inheritance patterns in larger populations.
Ultimately, the physical expression of seed shape, whether round or wrinkled, stems from specific sequences in the genetic code residing at specific chromosomal locations (loci). This simple observation emphasized how genetic variation leads to visible differences, further solidifying the core concepts that Mendel developed to help understand molecular genetics. His insights, though initially overlooked, have been remarkably influential, and the fundamental observations of seed shape continue to inform the study of genetics in many different organisms to this day.
Independent Assortment Breaking Down Mendel's 7 Key Cross-Breeding Experiments with Pea Plants - Pod Color Study Following Green and Yellow Pod Development
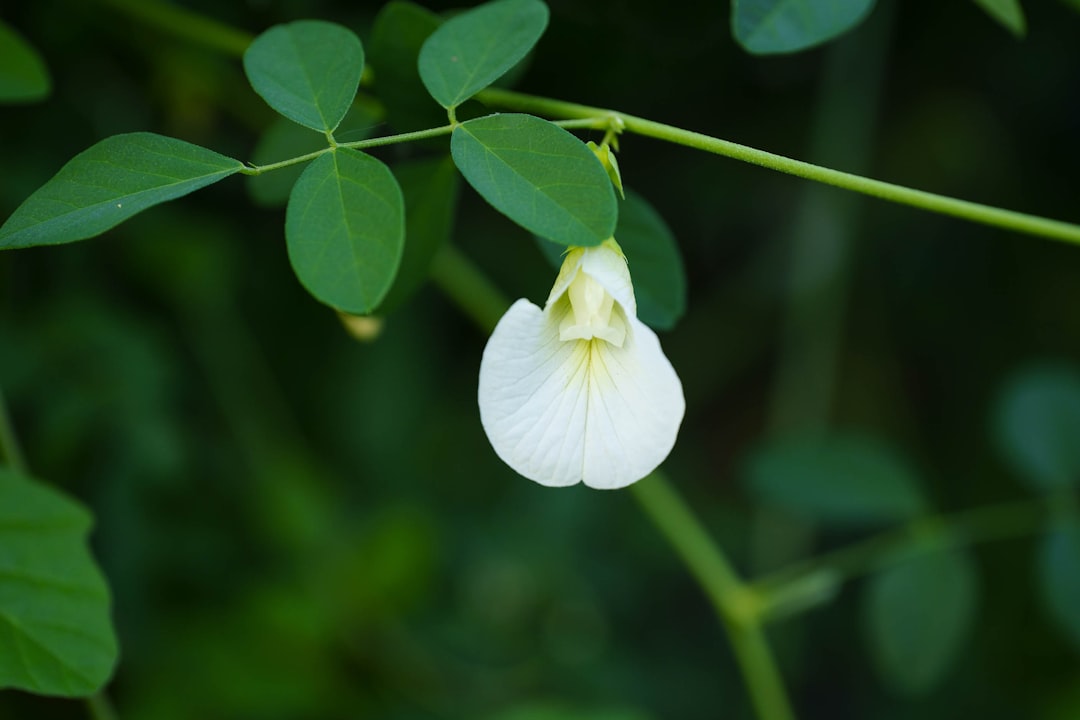
Mendel's investigation into pod color in pea plants further illustrates the principles of inheritance, specifically the dominance of green pods over yellow pods. This suggests a single gene controls pod color, where the green allele acts as the dominant form. Through his breeding experiments, Mendel established predictable inheritance patterns for pod color, supporting the concept of independent assortment in determining trait combinations in offspring. While modern research has located the gene responsible for pod color on chromosome 3, it's noteworthy that three of the traits Mendel originally studied still haven't been definitively linked to specific genes. This reinforces that the genetic basis for many traits remains complex and likely involves interactions between genes, as well as other factors influencing phenotypic expression. These remaining unknowns emphasize that Mendel's legacy continues to inspire ongoing research into the intricacies of inheritance.
Following Mendel's exploration of seed shape, specifically the round versus wrinkled characteristic, we can delve into the study of pod color. This involves understanding the inheritance patterns of green and yellow pod development.
Similar to seed shape, pod color appears to be controlled by a single gene, where green is dominant over yellow. We can trace this back to the genetic makeup of the plant. If a pea plant possesses at least one allele for yellow pods, it will display this trait. Conversely, green pod color only becomes apparent when the plant inherits two green pod alleles.
This aligns with Mendel's principle of independent assortment. The inheritance of pod color appears to happen independently of other traits like seed shape or flower color. For instance, during the formation of reproductive cells (gametes), the alleles controlling pod color separate and recombine randomly. This ensures that the inheritance of pod color does not directly impact the inheritance of seed shape or any other trait. This underscores the idea that traits are not necessarily linked, a concept that has significant implications for understanding the diversity of offspring in breeding experiments.
To further examine pod color mechanisms, researchers have employed various techniques, including comparative analysis of the transcriptome and metabolome. These studies help illuminate the biochemical and molecular underpinnings of pod coloration. The results provide a more in-depth understanding of the genes and proteins involved in pod color expression, which is useful for improving breeding programs aimed at modifying the color of pea pods.
It is also worth noting that despite intensive research on pod shape and color, along with other traits, scientists haven't yet identified the specific genes responsible for three of Mendel's seven characteristics. This highlights that while some aspects of pea plant genetics have been well-characterized, a considerable degree of uncertainty still exists. As our understanding of plant genetics progresses, it is possible that we will shed light on the remaining unresolved genetic components.
Just as in the seed shape investigation, pod color is influenced by the presence or absence of certain alleles and this is a critical part of how we think about plant inheritance today. However, it is crucial to keep in mind that this is just the tip of the iceberg for genetic complexity. The study of pod color can be an entry point into the world of gene expression and regulation, and it has been useful in providing a simpler context to explain more complicated scenarios that are likely to occur in the future.
Independent Assortment Breaking Down Mendel's 7 Key Cross-Breeding Experiments with Pea Plants - Pod Shape Investigation Recording Inflated vs Constricted Traits
Mendel's experiments with pea plant pods revealed a clear distinction between inflated and constricted pod shapes, where inflated was the dominant trait. This observation further solidified his understanding of dominant and recessive inheritance patterns. Crucially, his pod shape investigation provided more evidence for the idea of independent assortment. This means the inheritance of pod shape appeared to be entirely separate from other characteristics like seed shape or flower color. The consistent patterns observed in his breeding experiments with these pods, demonstrating specific ratios of trait inheritance, further corroborated his previous findings.
While Mendel's research into pod shape provided a clearer understanding of inheritance, it also underscored the complexity of genetics. It is notable that even with his detailed experiments, some aspects of pod shape (and other traits) are still poorly understood at the genetic level. This reinforces the idea that genetics is a complex field with much still to be discovered about the interplay between genes and traits in organisms. Mendel's meticulous work with pod shape not only reinforced his key laws of inheritance but also contributed significantly to our ongoing understanding of genetic variation and the specificities involved in successful plant breeding. This foundation continues to inform the direction of genetic research today.
Mendel's exploration of pod shape in pea plants, specifically the distinction between inflated and constricted pods, reveals a fascinating interplay between genetics and the environment. While inflated pods are considered the dominant trait, meaning even a single allele for this shape can mask the recessive constricted shape, the actual expression of these traits can be influenced by various factors. It's important to acknowledge that the inheritance of pod shape isn't governed by a single gene but is likely a more complex interaction of multiple genes.
This complexity becomes even more apparent when we consider the potential ecological implications of these pod shapes. Inflated pods offer a distinct advantage by providing greater capacity for seed storage and likely facilitating dispersal, thereby enhancing seed survival. Constriction, conversely, may reflect an adaptation to more challenging environments where seed production and dispersal might need to be more conservative. It's plausible that this trait is a remnant of periods of environmental stress in pea plant evolutionary history.
Despite the clarity of the inflated/constricted dominance pattern, precisely pinpointing the specific genes responsible for these pod shapes remains challenging. This, in itself, underscores the complexity of even seemingly simple traits. While Mendel clearly laid the groundwork for understanding these traits using his classic dominant-recessive relationship insights, modern genetics continues to refine our understanding of the underlying mechanisms that drive their variation.
Similar to his other experiments, Mendel used his insights into pod shape to further develop his principles of segregation and independent assortment. By diligently analyzing the outcomes of cross-breeding experiments and employing a statistical framework, he was able to make predictions regarding the ratios of various pod shapes in subsequent generations. This is a critical feature of modern breeding practices that Mendel helped establish.
The pod shape studies also provide a concrete example of the concept of allelism – how different alleles interact and influence traits. This idea forms the foundation for exploring more complex genetic influences and demonstrates how variations in pod shape relate to the genetic makeup of the plants.
Furthermore, Mendel's emphasis on pure-breeding lines within his experiments was influential for the development of future breeding programs. His observations on how certain traits are passed from one generation to the next, while initially seeming simple, are integral to understanding crop improvement strategies used by modern agriculture. We can trace back a lot of modern agriculture practices to these original observations of Mendel.
While Mendel's findings regarding pod shape, and other traits, were foundational, it's important to note that these traits often have deeper, far more complex interactions than Mendel's original experiments could have detected. Understanding how pod shapes relate to genetic variations, and their potential influence on environmental adaptability, continues to be an area of research that helps to further Mendel's seminal discoveries. His work laid the groundwork for an entirely new way to think about inheritance and variation, and we continue to refine his observations through the use of modern tools.
Independent Assortment Breaking Down Mendel's 7 Key Cross-Breeding Experiments with Pea Plants - Flower Position Research Examining Axial vs Terminal Locations
Mendel's research into flower position in pea plants focused on the difference between axial and terminal flower arrangements. Axial flowers, located along the stem, were found to be dominant over terminal flowers, which grow at the very tips of the stems. When Mendel tracked the traits across generations, he found that the F2 generation consistently showed a roughly 3:1 ratio of axial to terminal flowers. This predictable ratio is a strong demonstration of Mendel's ideas about independent assortment, specifically how different traits (like flower position) separate from each other during the formation of reproductive cells. This process of independent assortment means traits are inherited independently of each other.
His experiments with flower position didn't just reveal basic inheritance patterns but also the importance of controlling how plants reproduce (cross-breeding). This control is critical for accurately observing and interpreting how inherited traits are passed from one generation to the next. Mendel's detailed study of flower positioning offers a valuable piece of the puzzle in understanding the foundation of genetics. His work demonstrates how seemingly simple patterns in the appearance of plants can reveal intricate details about how traits are passed down, showcasing both the straightforward and the more complex aspects of genetic inheritance in living things.
1. **Flower Placement and Pollination:** The location of flowers on a pea plant, whether along the stem (axial) or at the tips (terminal), significantly influences how often they're pollinated. Axial flowers, being more readily accessible to pollinators, likely have a reproductive advantage compared to those at the ends of branches. This difference in accessibility could impact how traits get passed on to the next generation, influencing genetic diversity.
2. **Genetic Location of Flower Position:** Research has shown that the genes controlling whether a flower grows axially or terminally are located in different places on the plant's chromosomes. This separation helps us understand the concepts of gene linkage and independent assortment. It's interesting to consider how these genes interact with each other, as well as the environment.
3. **Beyond Genes: The Role of the Environment:** Genetics isn't the only thing that decides where a flower grows. Environmental factors like the amount of light and the quality of the soil can also influence whether a plant develops more axial or terminal flowers. This hints at the intricate relationship between a plant's genetic makeup and its external environment. It's a reminder that simply knowing the genes isn't sufficient to understand how a trait manifests.
4. **Evolutionary Benefits of Axial Flowers:** It seems plausible that axial flower placement might have evolved because it gives plants a better chance of survival. Being closer to the center of the plant potentially provides them with more sunlight and less obstruction from leaves, helping with photosynthesis and overall plant health. This makes me wonder what selective pressures in the past could have led to the predominance of this trait.
5. **Pollinator Attraction and Axial Flower Position:** Research suggests that axial flowers tend to draw more pollinators than terminal ones. This increase in pollination rates can lead to more cross-breeding and a higher rate of genetic mixing. I am curious about how this might impact the adaptation of pea plants to different environments, and whether it's an advantage in specific ecological niches.
6. **Mendel's Insights, Even in Side Observations:** Although Mendel's primary focus wasn't flower position, he diligently documented it in his studies. His meticulous approach reveals how seemingly minor details can provide valuable clues about the broader principles of genetics. It makes one appreciate the value of careful observation even for apparently minor characteristics.
7. **Phenotypic Plasticity: Genetics and Environment Intertwined:** The way a plant's flowers are arranged (axial or terminal) illustrates the concept of phenotypic plasticity. This means the genetic potential for certain traits can be molded by environmental influences. Understanding how these traits change based on external conditions can improve plant breeding for specific traits or contexts.
8. **Connecting Flower Position to Other Plant Traits:** Flower position is often studied alongside other traits, like plant height. This interconnectedness indicates that different traits might be controlled by genes that are closely linked or related through complex pathways. Understanding these relationships is important for developing more comprehensive models of plant breeding.
9. **The Struggle for Acceptance of New Ideas:** Initially, many scientists didn't see flower position as a particularly important trait in Mendel's time. The eventual recognition of its significance underscores a wider issue in science – the challenges of gaining acceptance for novel findings, especially those that challenge established views.
10. **Modern Genetic Techniques Illuminate Flower Positioning:** Today, researchers have powerful new genetic tools like advanced mapping techniques and molecular biology methods. These tools allow them to pinpoint specific genes responsible for flower placement, helping to connect Mendel's initial observations to modern-day genetic research and breeding applications. It’s remarkable how the foundation laid by classical genetics continues to be refined and expanded with the introduction of newer tools.
Independent Assortment Breaking Down Mendel's 7 Key Cross-Breeding Experiments with Pea Plants - Seed Color Documentation Tracking Yellow and Green Inheritance
Mendel's research into seed color focused on the inheritance patterns of yellow and green peas. Yellow seeds consistently demonstrated dominance over green, showcasing a clear pattern of inheritance. This dominance is evident in the offspring of both the first (F1) and second (F2) generations, where the presence of at least one dominant yellow allele leads to yellow seed expression. His meticulous tracking of phenotypic ratios within these generations solidified the concept of independent assortment, indicating that the traits for seed color separate independently during the formation of reproductive cells. These experiments underscored the strength of genetic dominance and provided a framework for understanding how traits are passed from one generation to the next. Mendel's work on seed color not only advanced our understanding of pea plant genetics but also laid the groundwork for future investigations into inheritance, highlighting the complexities and variations that can arise in trait combinations within offspring. While seemingly simple, this foundational research proved crucial in establishing the basic principles of genetics.
Mendel's exploration of seed color in pea plants revealed a straightforward dominance relationship where yellow seed color is dominant over green. This trait is controlled by a single gene, and the presence of at least one dominant yellow allele results in the yellow seed phenotype. This, while seemingly simple, provides a foundation for understanding the concept of genetic dominance, even if the underlying genetics are much more complex. Interestingly, the gene responsible for seed color resides on a particular chromosome, a discovery that provides a framework for understanding how traits are inherited and sorted during cell division.
However, the story isn't that simple. The yellow seed phenotype is associated with not only color but also other characteristics like seed shape and overall plant vigor. This phenomenon, known as pleiotropy, highlights how a single gene can affect multiple traits, which can complicate our attempts to predict inheritance patterns. Despite these complexities, Mendel's quantitative approach yielded predictable outcomes, particularly the 3:1 ratio of yellow to green seeds in the second generation (F2). This ability to predict ratios through statistical means has been foundational to modern genetic experiments and plays a role in understanding the frequency of traits in population genetics.
The inheritance of seed color isn't isolated. It interacts with other traits, like seed size or how well the seeds germinate. Comprehending these interactions is critical for breeding programs which aim for improved crops with multiple desirable traits, highlighting the importance of viewing the genetic landscape of plants in a holistic way. Furthermore, Mendel’s findings met initial resistance from his contemporaries, reminding us that groundbreaking ideas can take time to gain widespread acceptance. This resistance, however, only underscores the importance of thoroughly documented and robust experimentation.
Beyond the basic genetics, seed color is also susceptible to genetic drift, especially in smaller populations, where certain alleles may become more prevalent over time due to chance alone. The environment also plays a role. While yellow is genetically dominant, soil nutrient levels can influence how the seed color phenotype is expressed, making precise predictions about seed color challenging.
The implications of Mendel’s findings extend into today's world of plant breeding, where his insights form a core principle. Breeders use these inheritance patterns to select plants with desired traits, leading to more efficient and targeted breeding programs. Even today's gene modification techniques, which allow for a precise manipulation of genetic traits, have their roots in Mendel’s foundational observations of seed color. This enduring legacy illustrates the power of meticulous observation and fundamental research and how they can continue to shape the field of genetics and breeding well into the future.
Create AI-powered tutorials effortlessly: Learn, teach, and share knowledge with our intuitive platform. (Get started for free)
More Posts from aitutorialmaker.com: