Create AI-powered tutorials effortlessly: Learn, teach, and share knowledge with our intuitive platform. (Get started for free)
Unlocking the Secrets of Homogeneous Mixtures A Deep Dive into Uniform Chemistry
Unlocking the Secrets of Homogeneous Mixtures A Deep Dive into Uniform Chemistry - Understanding the Uniform Composition of Homogeneous Mixtures
The consistency of homogeneous mixtures lies in their uniform composition. Imagine a glass of saltwater – no matter where you take a sample from, it will always have the same ratio of salt to water. This is because the components are perfectly blended, unlike heterogeneous mixtures where you might see distinct parts or layers.
The seamless integration of components in homogeneous mixtures can lead to confusion with pure substances, since both appear uniform. However, the difference lies in the number of components present. While pure substances contain only one type of molecule, homogeneous mixtures contain multiple substances blended together, creating a single, homogeneous phase.
The concept of "like dissolves like" applies to these mixtures, guiding us in understanding why certain substances dissolve in others. For example, sugar dissolves in water, because both are polar molecules, allowing them to interact readily. Ultimately, understanding the uniform composition of homogeneous mixtures is key to appreciating their fundamental differences from heterogeneous mixtures and pure substances.
The uniformity of homogeneous mixtures is a fascinating aspect of chemistry. It's easy to assume that a substance with a consistent appearance must be a pure substance, but that's not always the case. Even though homogeneous mixtures contain multiple components, they're indistinguishable to the naked eye because those components are evenly dispersed at the molecular level. Air, for instance, is a prime example – a blend of gases like nitrogen and oxygen, mixed uniformly but not chemically bound.
The homogeneity of a mixture isn't always static. It can be influenced by factors like pressure and temperature, which can alter the molecular behavior and affect how uniformly components are distributed. This highlights the dynamic nature of homogeneous mixtures.
Despite their seemingly simple nature, homogeneous mixtures can be complex. Individual components often retain their original properties, leading to unique characteristics that can be leveraged in engineering. Solutions, a specific type of homogeneous mixture, are crucial in fields like pharmaceuticals, where consistent concentration of dissolved substances is critical for accurate dosing.
Separating components in a homogeneous mixture often requires specialized techniques like distillation or chromatography, revealing the intricate processes underlying their seemingly simple appearance. The concept of "like dissolves like" is a guiding principle for understanding how solvents and solutes interact in these mixtures. Their polarities and functional groups dictate their compatibility and ultimately the formation of the homogeneous mixture.
There are even more complex cases, like colloidal systems. These are a subtype of homogeneous mixtures where particles remain suspended within the medium, defying traditional notions of mixture stability. Some homogeneous mixtures can exhibit non-Newtonian fluid properties, further adding to their complexity and highlighting the constant need for deeper investigation.
The concept of homogeneity extends beyond chemistry. Materials science, for example, relies on uniform properties in alloys and composites to meet specific performance criteria. This concept highlights the importance of understanding homogeneity not only in chemical systems but also in various engineering disciplines.
Unlocking the Secrets of Homogeneous Mixtures A Deep Dive into Uniform Chemistry - Exploring the "Like Dissolves Like" Principle in Solution Chemistry
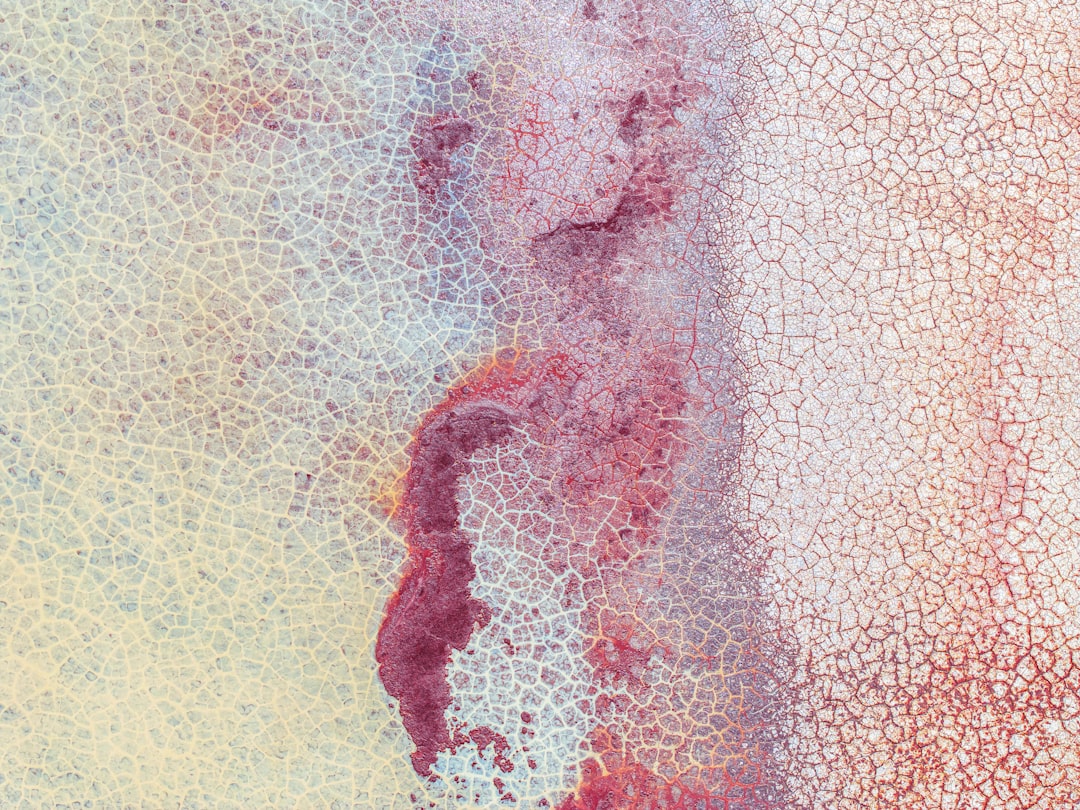
The "Like Dissolves Like" principle is a foundational concept in solution chemistry. It essentially states that substances with similar chemical properties tend to dissolve in each other. This is driven largely by the concept of polarity, which dictates how molecules interact. Polar solvents like water easily dissolve polar solutes like sugar and salt, creating homogeneous mixtures. Nonpolar solvents, in contrast, work best with nonpolar solutes like oils.
However, the "like dissolves like" principle is not without its exceptions. When dealing with ionic compounds, for example, the principle becomes more complex as these substances may dissociate into individual ions when dissolved in a polar solvent. Therefore, understanding the "like dissolves like" principle is crucial for comprehending the underlying dynamics of solubility, and its wide applications in various fields, including organic chemistry and materials science.
The concept of "like dissolves like" is a cornerstone of solution chemistry, guiding our understanding of why some substances dissolve readily in others while others remain stubbornly insoluble. The principle rests on the idea that molecules with similar polarities tend to mix well, while those with contrasting polarities are likely to separate. This makes sense when you consider how molecules interact – polar molecules, like water, are attracted to other polar molecules, forming strong hydrogen bonds. Nonpolar molecules, like oils, rely on weaker van der Waals forces for their interactions.
While this simple rule is a good starting point, there are numerous nuances and exceptions that make it essential to consider additional factors. Hydrophobic interactions, for example, can lead to surprising behaviors, especially in biological systems where molecules like lipids prefer to cluster together, avoiding contact with water.
Additionally, the size and structural complexity of molecules can also play a significant role in solubility. Even if two substances share similar polarities, a large molecule might not dissolve well due to steric hindrance, where the bulky structure of the molecule gets in the way of forming strong interactions with the solvent.
The concept of "like dissolves like" is further complicated by the existence of supercritical fluids. These fluids exist at temperatures and pressures above their critical point, possessing properties that transcend typical polarity rules. For instance, supercritical carbon dioxide, despite being nonpolar, can dissolve both polar and nonpolar substances, defying conventional solubility classifications.
Temperature also plays a crucial role. For most solid solutes in liquid solvents, increasing temperature often enhances their solubility, a phenomenon frequently leveraged in industrial processes where controlled heating can optimize solute absorption. However, this trend is not universally applicable, and some solutes may exhibit decreased solubility with rising temperatures.
Furthermore, the dissolution process itself differs for ionic compounds, which break apart into ions in polar solvents like water, compared to covalent compounds, which often dissolve through molecular interactions. This distinction is critical for understanding reactions in various fields, including materials science and chemical engineering.
The presence and arrangement of functional groups in organic molecules also play a significant role in solubility. The introduction of a hydroxyl (-OH) group can dramatically alter a molecule's interaction with water compared to similar hydrocarbons lacking such groups.
In real-world scenarios, homogeneous mixtures are rarely as simple as a single solute and solvent. Many formulations contain additional components, such as stabilizers or additives, that can alter the overall solubility dynamics.
The "like dissolves like" principle has a significant impact on everyday products, from cleaning agents to food products. Emulsifiers, for example, enable the blending of oil and water phases in salad dressings by bridging the gap between polar and nonpolar components.
Engineers routinely leverage this principle in designing chemical processes, ensuring the proper selection of materials and solvents for efficient reactions, crystallization processes, and separation techniques. By understanding the factors that influence solubility, we can more effectively control and optimize chemical reactions and processes, leading to the development of innovative products and technologies.
The "like dissolves like" principle is a valuable tool in our quest to understand how solutions form and how different substances interact with each other. While not without its exceptions, it provides a foundational framework for exploring the fascinating world of homogeneous mixtures and their intricate behaviors.
Unlocking the Secrets of Homogeneous Mixtures A Deep Dive into Uniform Chemistry - Examining Common Examples from Saltwater to Steel Alloys
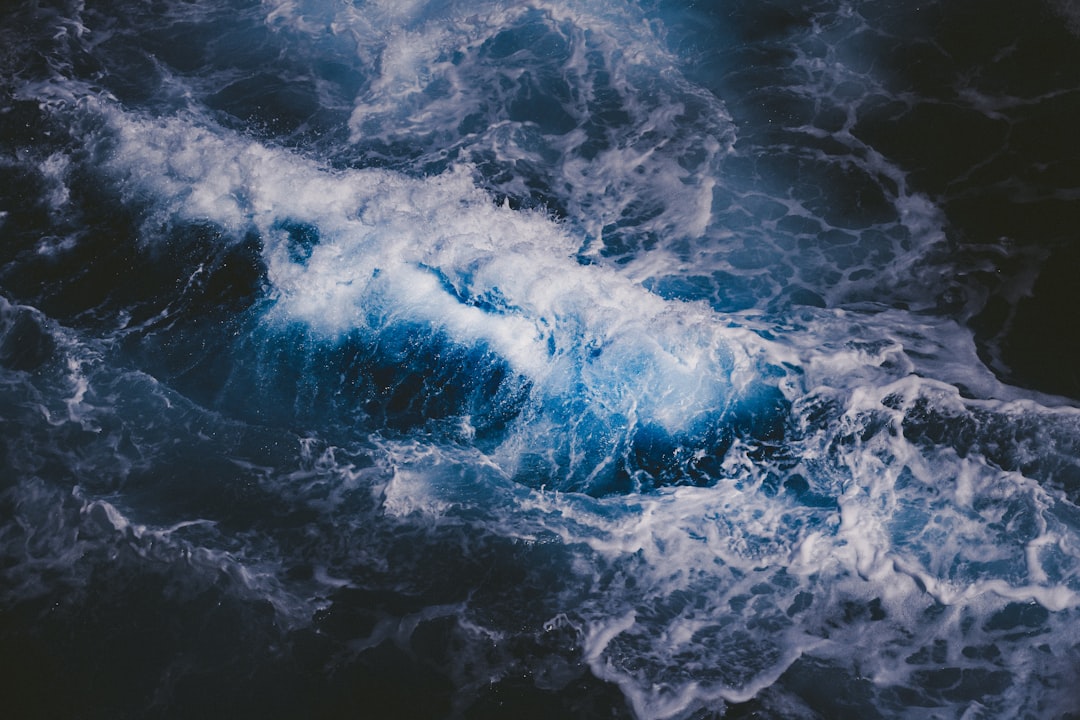
Let's delve into the world of homogeneous mixtures by looking at some everyday examples, from the familiar saltwater to the less obvious steel alloys. Saltwater, a classic example, demonstrates a uniform distribution of salt throughout the water. This uniformity means that regardless of where you take a sample, the salt-to-water ratio remains consistent. Turning to the realm of materials science, steel alloys exemplify the application of homogeneous mixtures. These alloys, often made up of iron combined with other metals like carbon, nickel, or chromium, are designed to have a consistent, uniform composition throughout. This careful balancing of components gives steel its specific properties, making it ideal for use in everything from construction to automobiles.
While many alloys showcase homogeneity, it's crucial to note that not all alloys fit neatly into this category. Some alloys can contain multiple phases, leading to variations in their composition and properties, blurring the lines between homogeneous and heterogeneous mixtures.
Examining these diverse examples – from the liquid world of saltwater to the solid realm of steel alloys – helps us appreciate the varied ways in which homogeneity manifests itself within the broader landscape of chemistry.
Examining homogeneous mixtures through specific examples from the realm of everyday experience can unveil their fascinating properties and intricate complexities. Let's delve into a few interesting cases, starting with saltwater.
Saltwater, though seemingly straightforward, isn't just a simple mixture. The dissolved ions significantly increase its density and boiling point compared to pure water, influencing marine life and requiring engineers to factor these properties into fluid dynamics calculations.
Moving on to steel alloys, the homogeneous blending of iron with other elements like carbon, manganese, and nickel allows engineers to precisely tailor their mechanical properties. For example, the strength and ductility of steel can be precisely controlled, creating materials suited for a wide range of applications.
Stainless steel's exceptional corrosion resistance isn't merely a result of surface treatment. It stems from the formation of a uniformly distributed, passive oxide layer directly attributed to its homogeneous composition. This highlights how seemingly simple mixtures can exhibit complex properties that significantly influence their performance.
The solubility of gases in liquids, such as oxygen in water, is another intriguing facet. This behavior is heavily influenced by external factors like pressure, underscoring how even homogeneous mixtures can demonstrate dynamic behavior under varying conditions. This insight is crucial for environmental science and fluid transport engineers.
Brass is an excellent example of an alloy formed by melting solid components. The homogeneity of this mixture is directly impacted by the cooling rate. The resulting microstructure, particularly the size of the microstructural features, influences the mechanical performance of brass, making it a crucial parameter in its engineering design.
Seawater, besides its uniform salt content, contains trace elements like calcium and magnesium. These elements, although present in minute quantities, significantly affect its conductivity. This knowledge is invaluable in developing sensors and monitoring systems for marine environments.
Ionic compounds like table salt readily dissolve in water to form homogeneous solutions. However, when dried, they crystallize back into distinct, heterogeneous structures, showcasing the dynamic nature of chemical states and the interplay between homogeneity and heterogeneity.
The interplay between solute and solvent in homogeneous mixtures can yield surprising outcomes. A sugar solution, upon heating, can reach supersaturation, where its concentration surpasses solubility limits without immediate crystallization. This phenomenon highlights the non-linear behavior that can occur within seemingly simple mixtures.
Creating homogeneous metal alloys often involves rapid cooling techniques, such as quenching, which trap non-equilibrium states. This allows engineers to generate materials with unique characteristics like increased strength or enhanced thermal stability.
Finally, colloidal systems, although appearing homogeneous, involve particles of significant size, often several hundred nanometers. These particles display fascinating behavior like Brownian motion, illustrating the nuanced interpretation of homogeneity at different scales.
These examples showcase the diverse characteristics and inherent complexity of homogeneous mixtures, demonstrating how seemingly simple mixtures can exhibit intricate behaviors influencing numerous fields, from engineering to marine science.
Unlocking the Secrets of Homogeneous Mixtures A Deep Dive into Uniform Chemistry - Distinguishing Between Pure Substances and Homogeneous Mixtures
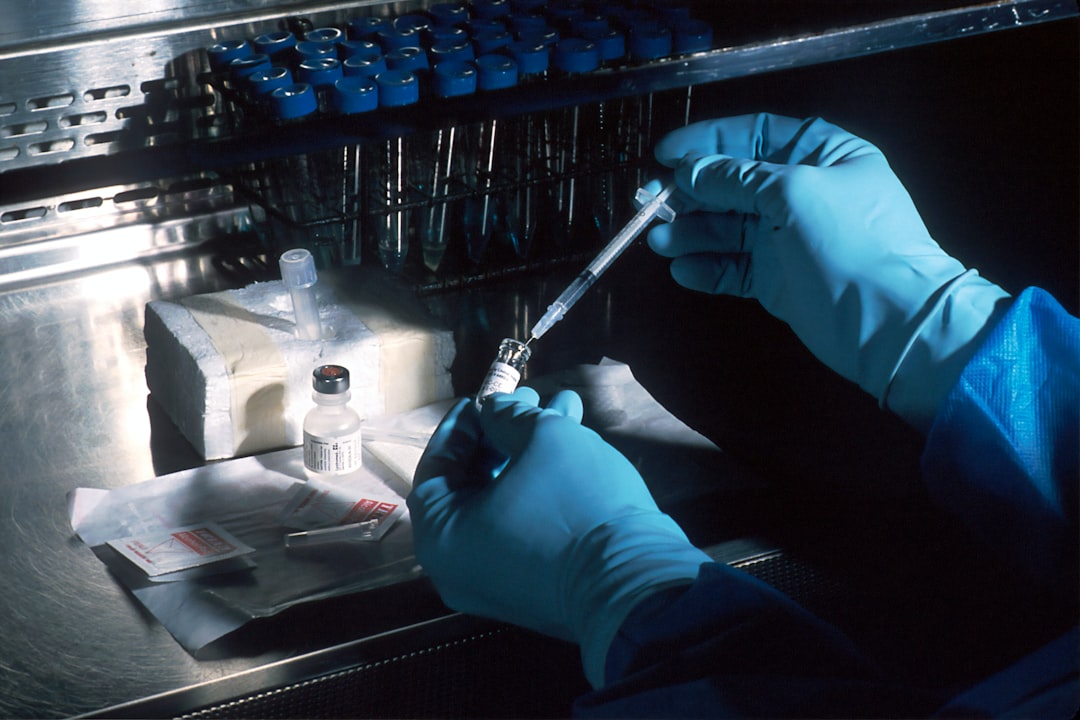
Distinguishing between pure substances and homogeneous mixtures is crucial in chemistry. Pure substances are single, unchanging components, like pure water or oxygen. Homogeneous mixtures, on the other hand, are blends of two or more substances uniformly mixed together. Imagine saltwater – every sip tastes the same because the salt is evenly distributed. This uniform nature makes homogeneous mixtures appear similar to pure substances. However, the key difference is that pure substances are composed of only one type of molecule, while homogeneous mixtures contain multiple substances blended seamlessly. Understanding this distinction is essential for various scientific studies and real-world applications in areas like materials science and chemical engineering.
Delving into the intricacies of homogeneous mixtures requires a keen eye for detail, as they often blur the line between simple mixtures and pure substances. The very definition of a pure substance, with its singular, unchanging composition, contrasts sharply with the multi-component nature of homogeneous mixtures, where components are seamlessly blended, often creating a single uniform phase. These distinctions, however, are not merely semantic exercises. They have profound implications for how we understand and apply materials in various fields.
Homogeneity transcends simple uniformity in appearance. Homogeneous mixtures, unlike their heterogeneous counterparts, can exist in a range of forms – solutions, colloids, and suspensions. This adds a layer of complexity to the classification of homogeneous mixtures, as different behaviors arise from these different structures. Even in homogeneous mixtures, individual components retain their chemical identity and properties, which is crucial in fields like pharmaceuticals where maintaining the functionality of each ingredient is paramount.
The seemingly effortless uniformity of homogeneous mixtures is an illusion, as their separation often requires specialized techniques like chromatography or centrifugation. These processes highlight the intricacies that underpin the deceptively simple appearance of homogeneous mixtures. The dynamic nature of these mixtures further complicates our understanding. Changes in temperature or pressure can disrupt the equilibrium of a homogeneous mixture. Increased temperature can alter the solubility of solutes in solutions or impact the solubility of gases in liquids, underscoring how external conditions influence material properties.
In the realm of engineering, homogeneous alloys are prized for their consistent properties, yet even these alloys can exhibit subtle variations in their microstructure, leading to complexities in their behavior. The understanding of these microscopic structures is critical for engineers who seek to predict and influence material properties like tensile strength and malleability. Supercritical fluids, existing in a state beyond the typical gas-liquid phase transition, further challenge our understanding of solubility. These fluids can dissolve both polar and nonpolar substances, enabling novel extraction techniques.
Colloids, though seemingly homogeneous, exhibit unique behaviors like the Tyndall effect and Brownian motion, emphasizing how homogeneity is often a matter of perspective and scale. The dissolution of ionic compounds into homogeneous solutions further complicates our understanding of homogeneity as it involves changes in microscopic interactions that lead to substantial changes in macroscopic properties like boiling and freezing points.
Organic chemistry introduces another layer of complexity by incorporating the role of functional groups. These groups can drastically alter solubility and reactivity in homogeneous mixtures, reminding us that even seemingly minor molecular differences can have significant impacts on chemical behavior. Understanding these intricacies is crucial for chemists and engineers who seek to control and optimize chemical reactions and processes.
The quest to unravel the secrets of homogeneous mixtures continues to be an exciting journey, as these seemingly simple materials exhibit an extraordinary range of behaviors that influence diverse applications, reminding us that the beauty of chemistry lies in its intricate complexities.
Unlocking the Secrets of Homogeneous Mixtures A Deep Dive into Uniform Chemistry - Analyzing the Single-Phase Nature of Uniform Mixtures
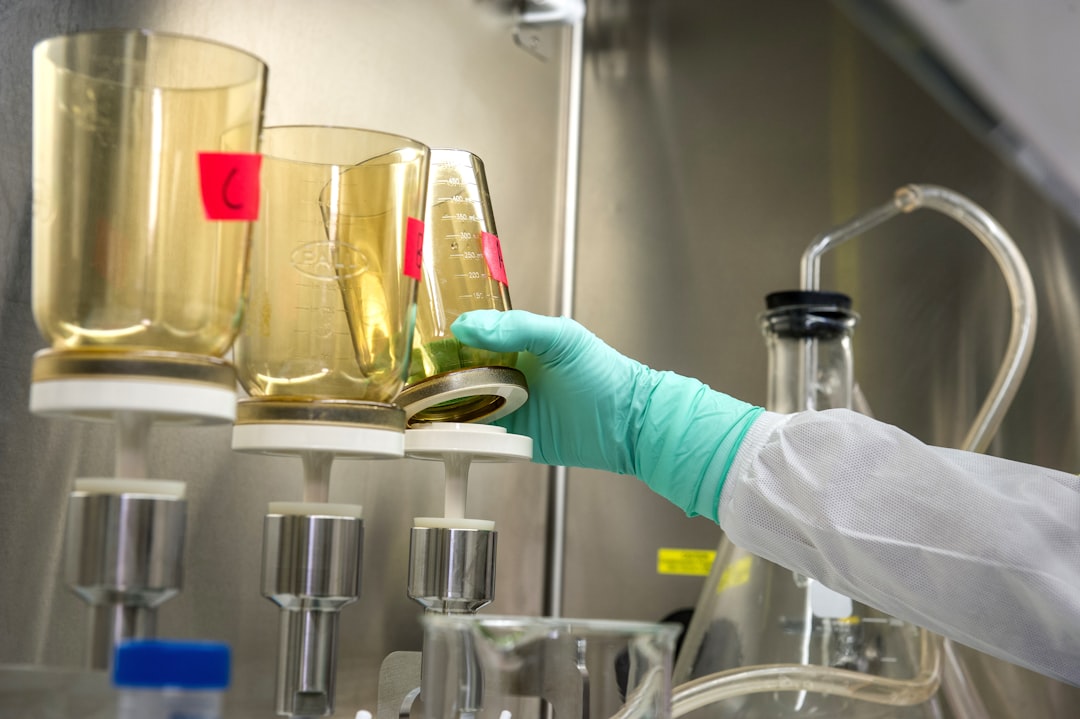
The single-phase nature of uniform mixtures, a defining feature of homogeneous systems, presents a fascinating challenge in understanding their complex behavior. These mixtures exhibit a uniform composition at the molecular level, making their components seemingly inseparable. Unlike heterogeneous mixtures with distinct phases, the components within homogeneous mixtures seamlessly blend together, creating a single, unified phase.
This uniform structure poses challenges in separating these components, highlighting their inherent complexity. Methods traditionally used for separating heterogeneous mixtures often fail when dealing with the consistent composition of homogeneous systems.
Examples like saltwater and certain alloys illustrate how the uniform distribution of elements within a single phase can lead to diverse and distinct properties. These properties have significant implications across a range of fields, from engineering, where specific properties are crucial in material selection, to environmental science, where understanding the behavior of dissolved substances in water is critical for monitoring and management.
Exploring the single-phase nature of homogeneous mixtures not only sheds light on their unique structure but also provides critical insights into their dynamic behaviors, particularly when subjected to changes in conditions such as temperature and pressure.
The single-phase nature of uniform mixtures implies a uniformity at the molecular level. This means that components not only blend evenly but also exist in a single, uninterrupted phase, with no distinct interfaces. This seemingly straightforward uniformity can have surprising consequences, like altering the boiling or melting points of the mixture compared to its individual components.
However, this homogeneity isn't static. Factors like temperature and pressure can significantly influence the balance of a uniform mixture. For instance, increased pressure can promote the dissolution of gases in liquids, a phenomenon crucial for industries like brewing or chemical processing. Even seemingly simple solutions, like sugar dissolved in water, can display complex behaviors such as supersaturation, where the concentration of dissolved solute exceeds the typical solubility limit without forming immediate crystals. This complicates our ability to predict how such mixtures will behave.
Colloidal systems, often categorized as homogeneous mixtures, introduce further complexity. These systems involve particles suspended within the medium, exhibiting unusual behaviors like Brownian motion. This contrasts with the typical notion of stable homogeneity and adds new dimensions to understanding these mixtures, particularly relevant in areas like drug formulation and food science.
The dissolution of ionic compounds, like salt in water, adds another layer of complexity. The ions dissociate and interact with the solvent, changing the solution's properties like conductivity and reactivity. This highlights the crucial role of microscopic interactions in shaping the behavior of seemingly simple solutions.
The solubility of substances in uniform mixtures isn't solely determined by polarity. Factors like molecular size and steric hindrance can also significantly impact interactions, demanding careful consideration in chemical engineering applications.
Homogeneous alloys, like stainless steel, exemplify how precise control over composition can directly influence the mechanical properties of materials. The resistance to corrosion and tensile strength of such alloys are crucial for engineering applications demanding safety and durability.
Supercritical fluids, operating beyond the conventional gas-liquid phase transition, provide a fascinating departure from typical solubility rules. They can dissolve a range of substances, despite differences in polarity, offering promising new techniques for extraction and separation in chemical industries.
Despite the appearance of stability, homogeneous mixtures can be quite dynamic. Changes in environmental conditions can trigger phase separation or alter solubility, influencing processes like crystal growth and separation technologies.
Some homogeneous mixtures even exhibit non-Newtonian fluid behavior, where viscosity changes under stress. This adds further complexity to designing and predicting material behavior in engineering applications, particularly in polymer solutions.
While we often perceive uniformity as simplicity, delving deeper into the nature of uniform mixtures reveals an intricate interplay of forces and factors influencing their behavior. Understanding these nuances is essential for unraveling the secrets of these fascinating systems and maximizing their applications in a wide range of fields.
Unlocking the Secrets of Homogeneous Mixtures A Deep Dive into Uniform Chemistry - Comparing Homogeneous and Heterogeneous Mixtures in Everyday Life
In everyday life, the difference between homogeneous and heterogeneous mixtures is readily apparent in their visual and structural properties. Homogeneous mixtures, like the vodka in your liquor cabinet, are uniform throughout. You won't see any distinct parts, as the ingredients are evenly distributed. Heterogeneous mixtures, however, are visibly non-uniform. Think of a salad, where you can clearly identify separate components like lettuce and tomatoes. This distinction between uniformity and non-uniformity extends beyond the kitchen, playing a critical role in fields like cooking and materials science, where the behavior of these mixtures can profoundly impact the final result.
Homogeneous mixtures, though seemingly simple, offer a treasure trove of fascinating complexity. While they present a uniform composition at a molecular level, their behavior can be surprisingly dynamic and nuanced.
Take water, often referred to as the "universal solvent." It forms homogeneous mixtures with a wide range of substances, a key factor in numerous industrial processes. But even water can display unexpected behavior when mixed with nanomaterials. The size and surface characteristics of these tiny particles drastically influence the mixture's properties like reactivity and strength.
We often see homogenous mixtures as static, but they're actually in constant dynamic equilibrium, which can be influenced by external factors. For instance, in gas-liquid solutions, increasing pressure can enhance gas solubility, a principle utilized in creating carbonated beverages.
Moreover, the thermal conductivity of homogeneous mixtures can vary drastically based on their composition. A metal alloy, for example, might conduct heat more efficiently than its individual components, crucial in applications like heat exchangers and electronic devices.
Even the viscosity of some homogeneous mixtures can be affected by stress, a phenomenon known as non-Newtonian behavior. This is particularly important in industries like food processing and cosmetics, where maintaining specific flow characteristics is vital for product consistency.
But the complexity of homogeneous mixtures doesn't end there. When ionic compounds dissolve in water, they dissociate into individual ions, altering the mixture's chemical properties like boiling point, melting point, conductivity, and reactivity.
Colloids, a type of homogeneous mixture, further challenge our understanding of homogeneity. These mixtures contain particles suspended in the medium, exhibiting unique behaviors like the Tyndall effect, where light scatters due to the presence of these particles.
Then we have supercritical fluids, a fascinating class of substances that defy traditional mixture definitions. They possess unique solvation characteristics, dissolving both polar and nonpolar substances, creating exciting opportunities for innovative extraction techniques in various industries.
Homogeneous mixtures can also exhibit non-linear behavior. Solutions, for instance, can become supersaturated, where a solute exceeds its typical solubility limit without crystallizing. This phenomenon has implications for crystal growth processes in industries ranging from drug formulation to materials synthesis.
Finally, even homogeneous alloys can exhibit microstructural variations, influencing their mechanical properties. These variations depend on factors like grain size and phase distribution, requiring engineers to precisely control processing conditions to achieve desired material characteristics.
The more we explore homogeneous mixtures, the more we uncover their intricate complexities, revealing a fascinating interplay of forces and factors that govern their behavior. Unlocking the secrets of these seemingly simple systems is crucial for advancing numerous fields, highlighting the continuous quest to understand the intricacies of our chemical world.
Create AI-powered tutorials effortlessly: Learn, teach, and share knowledge with our intuitive platform. (Get started for free)
More Posts from aitutorialmaker.com: